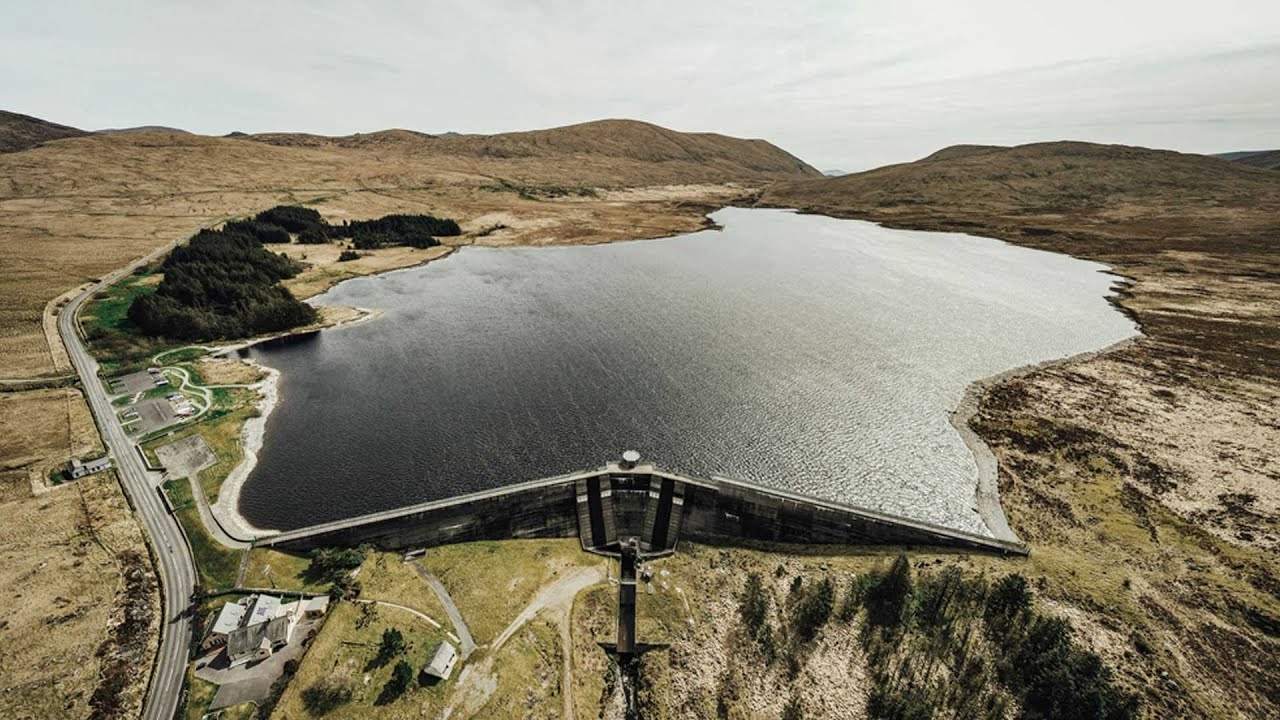
In December 2014, the reservoirs supplying water to the Brazilian city of São Paulo and 62 other cities in its state reached record low levels, leading to the most severe water crisis in the past 84 years. This scarcity had a direct impact on 15 million residents (three-quarters of its population), who experienced daily water restrictions lasting up to 12 hours. Fortunately, the situation improved with the return of rainfall.
It should encompass all available water sources, withstand the impacts of climate change, and ensure efficient management for the greater good. © Marcus Woodbridge / Unsplash
Four years later, in 2018, four million inhabitants of Cape Town, South Africa, were just days away from suffering what was announced as “day zero”: an absolute lack of water supply. Swift emergency measures averted the crisis.
Presently, in the metropolitan area of Barcelona, Spain, authorities are concluding exceptional emergency plans to address a water shortage from the Llobregat and Ter river basins. These basins primarily supply water to a conurbation of over two million people, and their reservoirs are currently at historically low levels.
Presently, in the metropolitan area of Barcelona, Spain, authorities are concluding exceptional emergency plans to address a water shortage from the Llobregat and Ter river basins. © David Gaya
In recent years, similar crises have occurred in Mexico City, Cairo, and the Indian cities of Chennai and Bangalore. All these cases have two critical factors in common: rapid population growth leading to a surge in water demand and diminishing rainfall in the reservoir basins and aquifers that serve as water sources. In some cases, there were specific factors. For instance, the visible issue in Mexico City and Cairo was insufficient investment in water infrastructure. Meanwhile, in Chennai and Bangalore, the persisting crisis stemmed from inadequate management of aquifer water, heavily reliant on the monsoon.
The Water Storage Gap
The water crises in these cities clearly illustrate the “storage gap.” This term refers to the disparity between the required amount of water and the operational storage capacity, which is crucial for making water accessible. Significantly, this factor goes beyond urban contexts and influences various essential services related to water, including irrigation, energy supply, and sanitation.
Freshwater storage can be broadly categorized into two main types: natural storage and purpose-built infrastructure. Natural storage encompasses glaciers, snowpacks, rivers, lakes, ponds, wetlands, floodplains, soil moisture, aquifers, and groundwater. On the other hand, purpose-built storage involves constructed features like reservoirs, sand dams, drainage canals, polders, underground dams, and “sponge cities” designed to tackle flooding and promote sustainable water management in urban areas.
Natural storage encompasses glaciers, snowpacks, rivers, lakes, ponds, wetlands, floodplains, soil moisture, aquifers, and groundwater. © wirestock/ Freepik
A recent World Bank report highlights a longstanding issue that has recently escalated. The water storage gap, identified decades ago, is expanding. This is due to the decline in natural storage and the aging of built storage, which outpaces efforts to rehabilitate or build new facilities. Compounding this challenge is the fact that the global population, having doubled over the last fifty years, continues to exert a growing demand for water resources.
In the last 50 years, there has been a notable reduction in freshwater storage by approximately 27 trillion cubic meters (m3), primarily attributed to the melting of global ice and the degradation of wetlands and floodplains. The Antarctic ice sheet alone has witnessed a loss of six trillion m3, while alpine glaciers and groundwater have decreased by eight trillion m3 and more than six trillion m3, respectively. Interestingly, the only increase in water storage has occurred in large dams, accounting for more than five trillion m3, along with small reservoirs, which have seen a rise of about one trillion m3.
Simultaneously, the demand for increased storage has surged due to significant shifts in population, reaching 8 billion in 2023 and the economic growth observed over the past five decades. The rapid rise in water demand aligns with a parallel increase in droughts attributed to climate change.
Towards a Paradigm Shift
UN-Water and the World Bank are championing a shift towards a fresh perspective on water storage that transcends narrow, local approaches and the exclusive emphasis on infrastructure development. They advocate for solutions that seamlessly incorporate natural storage. The envisioned approach must be systemic and inclusive, involving all sectors to ensure effective management. The central question is: Which combination of investments and policies can establish the most enduring and robust system for long-term water storage?
A significant challenge in adopting an integrated approach is redefining floods as a “surplus” water resource that can be harnessed and stored for periods of water scarcity. © Valuavitaly /Freepik
The initial emphasis should be on minimizing losses during transportation. Globally, non-revenue water (NRW) constitutes over 40% of drinking water, equating to around 45 million cubic meters per day, equivalent to 45,000 Olympic-size swimming pools. This represents water lost due to leaks, breakages, and errors—an amount sufficient to meet the needs of 200 million people. In certain African and Asian nations, the NRW rate surpasses 60%.
Collaborative water management involves recognizing that activities in one region can impact others. This is the case of the eternal dilemma between urban water supply, agricultural water needs, and environmental conservation, particularly evident in inter-basin transfers. Addressing this requires exploring various options, beginning with a comprehensive understanding of the existing storage system, emphasizing the capacities of natural storage that contribute to environmental well-being. A significant challenge in adopting an integrated approach is redefining floods as a “surplus” water resource that can be harnessed and stored for periods of water scarcity.
Inadequate management of water storage as a holistic system often leads to an excessive reliance on constructed storage, neglecting the benefits of natural storage. Ideally, if natural storage retains surplus water during dry seasons, built storage should tap into this resource. This approach is not only feasible but also represents the most effective means to ensure water security.